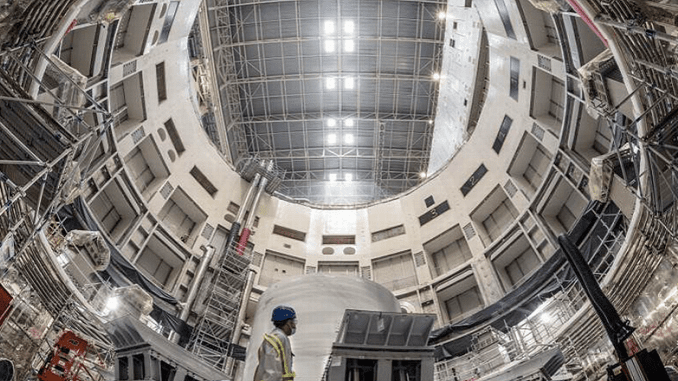
PARIS, France, November 1, 2021 (ENS) – In the tiny 10th century French town of Saint-Paul-lès-Durance in Provence, the largest scientific research collaboration in history is underway in the most expensive building ever built. The international nuclear fusion megaproject has brought all the countries that top the greenhouse gas emissions list together in an unprecedented global collaboration.
The Cadarache research center for nuclear energy, Europe’s largest, is located in Saint-Paul-lès-Durance. Right next door, 35 countries are creating the world’s largest experimental fusion facility, the International Thermonuclear Experimental Reactor, or ITER, designed to demonstrate the scientific and technological feasibility of fusion power. The target date for operation to start is 2035.
Like the Paris Agreement on climate, the ITER is also a first-of-its-kind global collaboration.
The 35 nations participating in ITER are the 27 European Union countries + (through Euratom) Switzerland, and the United Kingdom + China, India, Japan, South Korea, Russia, and the United States.
The ITER Organization has also concluded non-member technical cooperation agreements with Australia, through the Australian Nuclear Science and Technology Organisation; Kazakhstan, through Kazakhstan’s National Nuclear Center, and Canada.
The United Kingdom is participating in ITER post-Brexit through its membership in Fusion for Energy, the European Domestic Agency for ITER.
Taken together, ITER Member nations represent three continents, over 40 languages, half of the world’s population and 85 percent of global gross domestic product. In the offices of the ITER Organization and the domestic agencies, in laboratories and in industry, thousands of people are working toward the success of ITER.
Risks and Benefits of Nuclear Fusion
Completely different from the nuclear fission that splits atoms to power all current nuclear generating stations, fusion is the process that powers the Sun and the stars.
Fusion occurs when two light atoms bond together, or fuse, to make a heavier one. When light atomic nuclei fuse, a large amount of energy is released.
But the process requires temperatures far beyond what any solid material could withstand. To capture the Sun’s power source here on Earth, what’s needed is a way of capturing and containing something that hot – 100,000,000 degrees or more – by suspending it in a way that prevents it from coming into contact with anything solid, explains David Chandler of the Massachusetts Institute of Technology, MIT.
“That’s done through intense magnetic fields, which form a kind of invisible bottle to contain the hot swirling soup of protons and electrons, called a plasma,” Chandler writes. “Because the particles have an electric charge, they are strongly controlled by the magnetic fields, and the most widely used configuration for containing them is a donut-shaped device called a tokamak.”
Most of these devices have produced their magnetic fields using conventional electromagnets made of copper, but the ITER uses low-temperature superconductors.
Conceived as the last experimental step to prove the feasibility of fusion as a large-scale and carbon-free source of energy, ITER will be the world’s largest tokamak, with 10 times the plasma volume of the largest tokamak operating today.
A tokamak is a fusion device for containing a plasma inside a torus chamber through the use of two magnetic fields – one created by electric coils around the torus, the other created by intense electric current in the plasma itself. More than 200 tokamaks around the world have paved the way to the ITER collaboration.
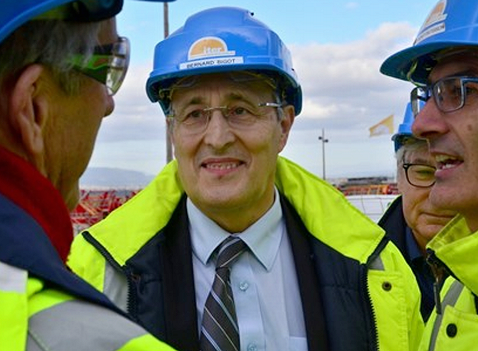
“It is because of hydrogen fusion that we are all alive today. Without fusion taking place in the Sun, we would have no light or warmth and there would be no light on Earth,” said ITER Director-General Bernard Bigot at the formal launch of the ITER tokamak assembly on July 29, 2020.
The ITER Tokamak will be the largest ever built, with a plasma volume of 840 cubic meters and a weight of 23,000 tons. The maximum plasma volume in current tokamaks is 100 cubic meters. When operational, ITER is expected to produce 500 MW of fusion power.
Bigot explained that not only would the ITER’s fusion reaction produce more energy more safely than nuclear fission reactors, it would also produce far less harmful radioactive waste than fission, from which weapons-grade material in spent fuel rods taking millions of years to decay requires extremely careful and expensive storage.
Fusion requires much less fuel than fission and the fuel is easier to obtain. Fission requires uranium that must be mined and enriched; fusion requires deuterium, readily extractable from seawater, and tritium, which can be made in the reactor itself from lithium.
ITER scientists estimate that, “Terrestrial reserves of lithium would permit the operation of fusion power plants for more than 1,000 years, while sea-based reserves of lithium would fulfil needs for millions of years.”
Fusion Reactors Don’t Melt Down or Emit Long-Lived Radioactive Waste
The type of uncontrolled nuclear meltdown that happened at Chernobyl or Fukushima nuclear power plants is just not possible at ITER or any other nuclear fusion facility, according to the International Atomic Energy Agency, IAEA.
“It is absolutely impossible for a Fukushima-type accident to happen at ITER,” the official documentation states. “The [fusion] reaction relies on a continuous input of fuel; if there is any perturbation in this process, the reaction ceases immediately.”
The waste produced by nuclear fusion is not long-lived as fission waste products are.
“A fusion reactor produces helium, which is an inert gas. It also produces and consumes tritium within the plant in a closed circuit. Tritium is radioactive (a beta emitter) but its half life is short. It is only used in low amounts so, unlike long-lived radioactive nuclei, it cannot produce any serious danger,” the IAEA explains.
But the IAEA does raise another possible safety challenge, saying, “The activation of the reactor’s structural material by intense neutron fluxes is another issue. This strongly depends on what solution for blanket and other structures has been adopted, and its reduction is an important challenge for future fusion experiments.”
The biggest disadvantage of nuclear fusion is apparent – it is very challenging to achieve. Nuclear fission power plants have been online since the 1950s, while fusion is still to be achieved at any great scale, although several dozen experimental fusion projects are underway around the world.
An Informal ITER Progress Report
The ITER Council meets twice a year. At its most recent meeting in June, the Council noted, with appreciation, the project’s achievements since its last meeting in November 2020, including the continued delivery of major components and progress in machine assembly, despite difficulties due to the coronavirus pandemic.
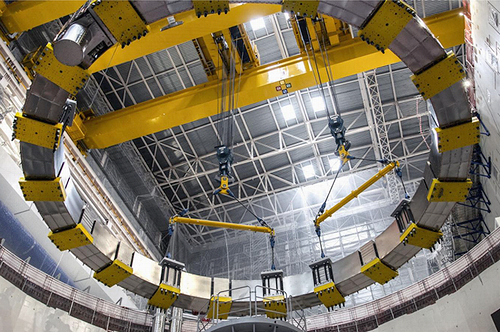
Here’s an informal progress report as of June 2021, when ITER Council made the latest data available:
• The first of ITER’s superconducting magnets, poloidal field coil #6, procured by Europe in collaboration with China, has been positioned in the tokamak pit, with one additional poloidal field coil completed and the others steadily progressing.
• Seven toroidal field coils have now been delivered to the ITER site, with the eighth completed and ready for shipment. The total of 18 toroidal field coils will produce a magnetic field around the ITER tokamak torus to confine the plasma particles.
• The first module of the central solenoid is fully qualified and now ready for shipment, with the second to ship later.
• Assembly of the first vacuum vessel sector sub-assembly, fabricated in Korea with port stubs supplied by Russia, has begun in the Assembly Hall, incorporating the associated toroidal field coils and thermal shield elements.
• All cryostat elements have been delivered to the ITER site, and welding is beginning on the cryostat lid. The cryostat lower cylinder supplied by India has been installed.
• Manufacturing of the other key components is underway in the Members’ industrial enterprises.
• Major progress has been achieved on plant systems for reactive power compensation, magnet power conversion, cryogenics, and cooling water, with multiple systems beginning or preparing for commissioning.
• US ITER will contribute hardware in support of 12 different ITER systems, including the central solenoid in the magnet system, used to induce the majority of the magnetic flux change needed to initiate the plasma, generate the plasma current, and maintain this current during the burn time.
The ITER Project readiness for the first plasma to enter the system is now approximately 75 percent complete.
Who’s Picking Up the Bill?
The European Union with Switzerland is contributing almost half of the costs of ITER construction, while the other six members of this joint international venture: China, India, Japan, South Korea, Russia, and the United States, are contributing equally to fund the rest.
Ninety percent of contributions will be delivered “in-kind.” In the place of cash, the Members will deliver components and buildings directly to the ITER Organization.
The ITER Organization estimates the cost of construction for the seven Members at roughly €13 billion, if all the manufacturing were done in Europe.
But each Member State is producing its contributions in its own country and delivering them to the ITER site in France. “As production costs vary from Member to Member, it is impossible to furnish a more precise estimation,” says the ITER Organization.
To manage production costs and country contributions, back in 2011 ITER established its own currency. ITER Units of Account, IUAs, are the currency, a unique feature of the ITER project based on the principle of procurement sharing. Instead of contributing cash to the project, the Members are providing “in-kind” contributions.
The IUA was devised in order to measure the value of in-kind contributions consistently over time, and neutralize market fluctuations.
ITER Is Not the World’s Only Tokamak
ITER is a first-of-a-kind global collaboration, an ambitious effort to advance fusion science and technology to the point where demonstration fusion power plants can be designed and built, but many other fusion experiments, too, are underway around the world.
At the Massachusetts Institute of Technology, MIT, on September 5, for the first time, a large high-temperature superconducting electromagnet was ramped up to a field strength of 20 tesla, the most powerful magnetic field of its kind ever created on Earth.
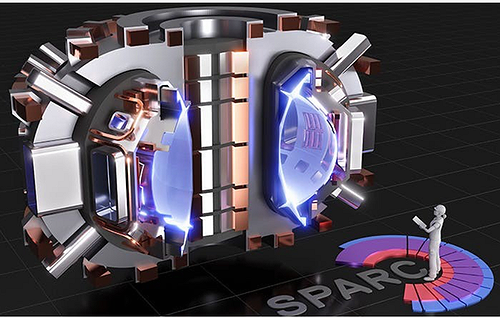
That successful demonstration helps resolve the greatest uncertainty in the quest to build the world’s first fusion power plant that can produce more power than it consumes, according to the project’s leaders at MIT and startup company Commonwealth Fusion Systems, CFS.
That combination of scientifically established design principles and game-changing magnetic field strength is what makes it possible to achieve a plant that could be economically viable and developed on a fast track.
“It’s a big moment,” says Bob Mumgaard, CEO of Commonwealth Fusion Systems. “We now have a platform that is both scientifically very well-advanced, because of the decades of research on these machines, and also commercially very interesting.”
The major innovation in the MIT-CFS fusion design is the use of high-temperature superconductors, which enable a much stronger magnetic field in a smaller space. This design was made possible by a new kind of superconducting material that became commercially available a few years ago.
The idea arose as a class project in a nuclear engineering class taught by Dennis Whyte, director of MIT’s Plasma Science and Fusion Center. The idea seemed so promising that it continued to be developed over the next few iterations of that class, leading to the ARC power plant design concept in early 2015.
Until then, the only way to achieve the colossally powerful magnetic fields needed to create a magnetic “bottle” capable of containing plasma heated up to hundreds of millions of degrees was to make them larger and larger.
But the new high-temperature superconductor material, made in the form of a flat, ribbon-like tape, makes it possible to achieve a higher magnetic field in a smaller device, equaling the performance that would be achieved in an apparatus 40 times larger in volume using conventional low-temperature superconducting magnets.
That leap in power versus size is the key element in ARC’s revolutionary design.
The use of the new high-temperature superconducting magnets makes it possible to apply decades of experimental knowledge gained from the operation of tokamak experiments, including MIT’s own Alcator series, now in safe shutdown.
With the magnet technology now successfully demonstrated, the MIT-CFS collaboration is on track to build the world’s first fusion device that can create and confine a plasma that produces more energy than it consumes. That demonstration device, called SPARC, is targeted for completion in 2025.
“The challenges of making fusion happen are both technical and scientific,” says Whyte. But once the technology is proven, he says, “it’s an inexhaustible, carbon-free source of energy that you can deploy anywhere and at any time. It’s really a fundamentally new energy source.”
Nearly every country now participating in the ITER project, and several others, has a homegrown nuclear fusion project of its own, and sometimes more than one.
The United States has four tokomaks besides the MIT SPARC project, Russia has four, China has three, the Czech Republic has two and so do Germany, Great Britain, and India, Switzerland has one and so do Brazil, Canada, Portugal, South Korea, and Japan.
For a list of all tokomaks worldwide, visit The Max Planck Institute for Plasma Physics. IPP is part of the European Fusion Programme within the framework of the European Consortium for the Development of Fusion Energy, EUROfusion. The consortium is coordinated by IPP in Garching, Germany and comprises 30 fusion centers from 26 countries of the European Union as well as Switzerland and Ukraine.
Taking It On the Road
EUROfusion, is premiering a traveling exhibition on fusion energy that aims to be both educational and participatory.
Fusion, Power to the People, which opened on October 8 in Marseille, France, combines science, art and technology to make a complex topic accessible to all kinds of audiences.
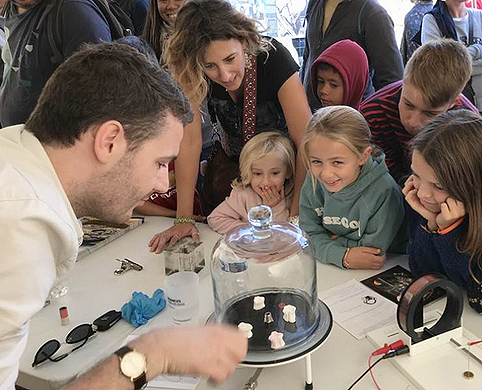
Hosted at Marseille’s historic Les Docks Village, the exhibition looks to the past, present and future to help visitors understand what powers the Sun and stars, and show the efforts being made to harness that potential as a new source of clean, abundant and safe energy.
Visitors will have the opportunity to download a mobile application for a truly interactive experience. Via the mobile app, visitors meet a cast of characters, are introduced to a story of science and suspense, and interact with the exhibition. By navigating different spaces and unlocking crucial information, visitors explore and experience the exhibition as a series of unique activities and discoveries.
The exhibition is open, free of charge, in Marseille through December 19. After Marseille, Fusion, Power to the People will travel across Europe.
“Fusion energy is not familiar to most, but we believe it’s a vital topic for people to explore,” says EUROfusion Outreach Officer Mohamed Belhorma who developed the traveling show, Fusion, Power to the People. “We created the exhibition to give a fun and engaging introduction to fusion energy. We want to show the public that fusion has the potential to be an important piece in solving the energy puzzle.”
Featured image: India’s contractor MAN Energy Solutions, a sub-contractor of cryostat segment manufacturer Larsen & Toubro, finishes welding the lower cylinder of the ITER cryostat to the base. The cryostat will completely surround the vacuum vessel and superconducting magnets of the ITER fusion machine, insulating the magnetic system at an ultra-cold temperature. The 3,800-tonne cryostat will be the world’s largest steel vacuum chamber. March 2021. (Photo courtesy ITER)